Authors: Deema Al Swidani, Evin Genc, August 26. 2024
1 Definition and Relevance
Chemical pollution is caused by the release of harmful chemical substances into the environment because of human activities. This includes both intentional and unintentional emissions such as exhaust gases, industrial waste and pesticide residues.[i] Chemical pollutants come from a variety of sources, including industrial processes, agricultural practices and the daily use of household products. These often have a negative impact on air, water and soil.1 Chemical pollution has an enormous negative impact on human health and the environment. The threat posed by chemical pollution is far-reaching and complex, as not only the individual chemicals but also their interactions in the environment can have toxic effects.[ii] In industry, pollutants such as heavy metals, solvents and organic chemicals are produced as by-products of manufacturing. In agriculture, pesticides, herbicides and fertilizers end up in the soil and groundwater, which contributes to environmental pollution.[iii] In addition, vehicle exhaust fumes and emissions from power plants contribute significantly to chemical pollution.
According to forecasts, chemical production is expected to be three times higher by 2050 than it is today. Global chemical production has already increased by a factor of 50 since the 1950s, highlighting the need for strict monitoring and control of chemical pollution.[iv] Of particular concern is the increasing production of chemicals in emerging economies, where environmental and health regulations are often less stringent than in industrialized countries. This trend reinforces the urgency to strengthen international standards and cooperation to minimize the impact of chemical pollution on global environmental and health conditions.
Chemical pollution can be divided into two main categories: Organic pollutants and inorganic pollutants. Persistent organic pollutants (POPs) include substances such as per- and polyfluoroalkyl substances (PFAS), which are particularly problematic due to their difficulty in biodegrading and their tendency to accumulate in the environment and the human body. These pollutants, which arise both through deliberate production and as unintentional by-products, are widespread in heavily industrialized regions. [v] POPs accumulate in the food chain and pose a serious risk to humans and the environment due to their toxic properties, persistence and ability to bioaccumulate. Despite international action under the Stockholm Convention, which since 2001 has laid down global rules to reduce and ban POPs, the problem remains, particularly in countries where these substances are still in use, where they pose a serious environmental and health risk.[vi] Heavy metals, nutrients, sediments and industrial waste are common inorganic pollutants that are steadily rising in concentration due to human activities like mining and agriculture. Pollutants such as arsenic, lead and mercury are frequently generated as by-products and build up in the environment, resulting in harmful effects on soil, water and living organisms.[vii] Over time, this accumulation can lead to significant damage to health and the environment.
The most common chemicals that contribute to environmental pollution include the following substances, which can be seen in Table 1:
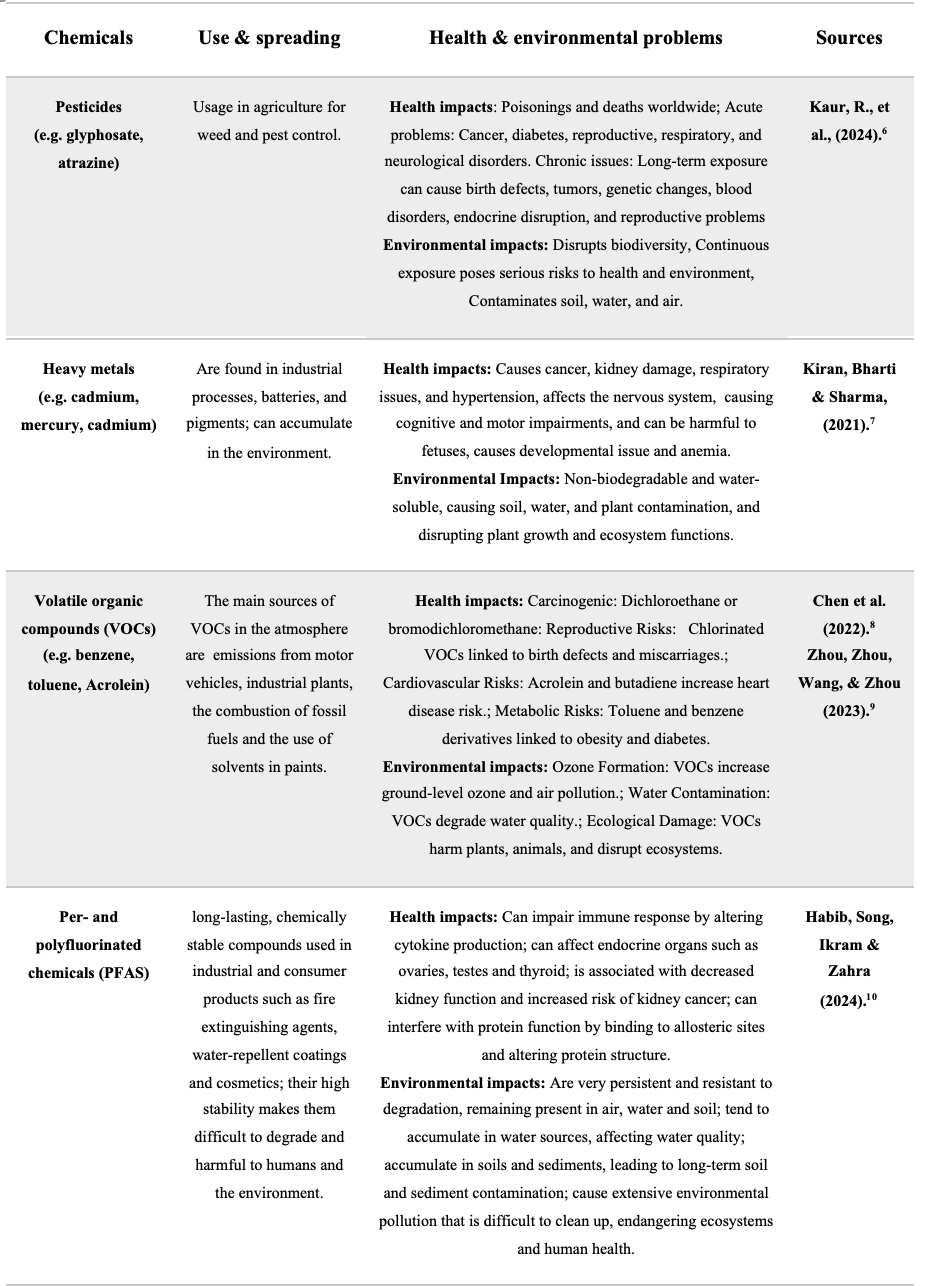
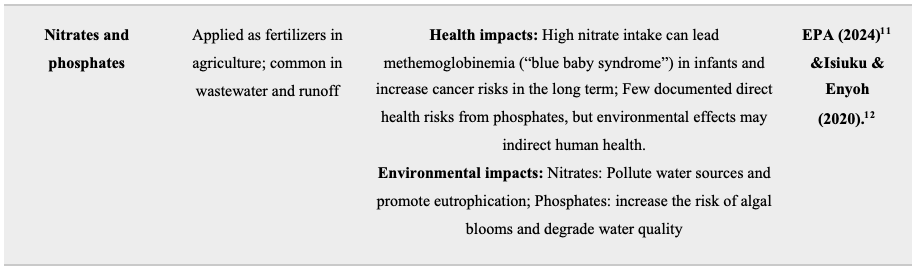
According to Persson et al. (2022), chemical pollution poses a serious global threat as it persists in the environment, can accumulate in living organisms and is capable of being transported over long distances through different ecosystems.[xv]These characteristics highlight the persistent and widespread nature of many synthetic chemicals and emphasize the need for strict global monitoring and regulation. In addition, Rockström et al. (2009) calls for the setting of limits on chemical pollution to protect the planet and identifies pollutants and heavy metals as key indicators for these limits. The authors point out that such limits must be determined by societal decisions to assess risks and uncertainties. This means that we need to determine what amount of pollution is still considered safe and how we deal with the associated risks.[xvi] In addition to these findings, it is crucial that we develop targeted and effective pollution reduction measures to comply with the defined planetary boundaries. Only through close international cooperation and continuous monitoring can we ensure that environmental impacts are minimized, and the long-term stability of our ecosystems remains guaranteed.
2 Influence of firms on chemical pollution
The chemical industry is the second-largest manufacturing sector in the world and is currently worth more than 5 trillion US dollars per year.[xvii] Companies, especially those in the chemical industry, are largely responsible for chemical pollution. With annual emissions of around 2 billion tons of carbon dioxide (CO2), the chemical industry contributes significantly to global pollution, accounting for around 5% of global greenhouse gas emissions.[xviii] The chemical and petrochemical industry is one of the most energy-intensive sectors and predominantly uses fossil fuels as its primary source of raw materials, which significantly increases its environmental impact. The sector is also the largest industrial energy consumer worldwide.[xix] The chemical industry is not only a factor in high energy consumption, but also an important player in energy conservation. Chemical products and technologies are used in numerous applications that either increase energy efficiency or enable the use of renewable energies.19 This dual function of the industry shows that it cannot only contribute to the problem, but also to solving global energy challenges.
The role of companies in chemical pollution is particularly important. They are not only responsible for producing chemicals, but also for their use and disposal. Industrial processes contribute significantly to pollution, particularly in emerging and developing countries, where environmental regulations are often less stringent. One example is the textile industry. The textile industry contributes significantly to industrial wastewater pollution, with up to 20 % estimated to be attributable to dyeing and finishing processes.[xx] Chemical pollution is a significant environmental problem that is being exacerbated by the rapid growth of the fast fashion industry. Textile companies are responsible not only for the production of clothing, but also for the entire life cycle of their products, including their disposal. Improper disposal practices such as landfilling or incineration release harmful chemicals and greenhouse gases into the environment. Companies also have a responsibility to adopt sustainable practices that reduce waste production and promote recycling. Without such measures, the environmental impact will continue to increase and exacerbate existing environmental challenges.
Chemicals are used in various sectors, such as agriculture and the production of consumer goods, and contribute significantly to environmental pollution. Pesticides and fertilizers lead to soil and water contamination, which endangers both soil quality and health.[xxi] However, companies also have the opportunity to contribute to the reduction of chemical pollution through initiatives such as ”Green Chemistry” by minimizing the environmental impact of chemicals. This includes reducing dependence on fossil fuels and efficiently converting renewable raw materials into chemicals, as well as minimizing the environmental impacts and risks of chemical manufacturing processes.[xxii] Strict adherence to environmental standards and clean production technologies are crucial to effectively tackle the challenges of chemical pollution. In summary, it can be said that companies, especially in the chemical sector, contribute to both the creation and control of chemical pollution. Their responsibility in reducing the environmental impact of chemical emissions is central to a sustainable future.
2.1 Effects of chemical pollution on the environment and health
Chemical pollution is one of the most serious threats to the environment and health worldwide. The effects of this pollution are felt both directly and indirectly and have an impact on a wide range of environmental and health problems. One major problem is eutrophication, which is caused by the excessive use of nitrogen fertilizers in agriculture. These fertilizers enter bodies of water via surface run-off and lead to oversaturation with nutrients. Dead zones are created, which have serious negative impacts the loss of biodiversity, damage to the fishing industry, disruption to marine food webs and a deterioration in water quality. The Gulf of Mexico is particularly badly affected, where over 20,000 square kilometres of dead zones are created every year, mainly due to agricultural nutrient run-off.[xxiii]
Another example that illustrates the negative effects of chemical pollution is the drastic decline in vulture populations in India caused by the drug diclofenac. This non-steroidal anti-inflammatory drug, which is used in veterinary medicine, led to massive poisoning of vultures that consumed the carcasses due to residues in them. In some regions, vulture populations shrank by more than 90%, endangering not only the vulture species themselves but also the entire ecological balance.[xxiv] The decline of vultures has an enormous impact on the entire ecology.
Chemical pollution also has enormous negative effects on health. Pollutants such as lead, mercury and pesticides contribute to serious health problems worldwide.. It is estimated that millions of people worldwide die every year from the effects of chemical pollution, mainly caused by pollution of air, water and soil. These pollutants are responsible for a variety of diseases, including respiratory diseases, neurological disorders and hormonal imbalances. Exposure to lead can be particularly dangerous to human health. Lead can originate from various sources, including industrial processes, lead-based paints, old lead pipes, and contaminated dust. According to the World Health Organization (WHO), people are especially at risk from inhaling lead particles and ingesting lead-contaminated dust, water, and food.[xxv] A 2020 study found that high blood lead concentrations were associated with an increased risk of cardiovascular diseases and higher mortality. Over a median follow-up of 19.3 years, 4,422 participants died, with 38% of these deaths attributed to cardiovascular diseases. These findings underscore that even low levels of lead can pose serious health risks.[xxvi]
The economic burdens of chemical pollution are enormous. According to the European Environment Agency, PFAS comprise over 4,700 chemicals. Due to the great variety and the different areas of application of the chemicals used in Europe, it is currently not possible to carry out comprehensive environmental and health risk assessments for all substances.[xxvii] For example, the Royal Society of Chemistry has urged the UK government to tighten drinking water standards, noting that over a third of water bodies in England and Wales show high PFAS risk levels. They highlight that these persistent chemicals, commonly found in everyday products, pose serious health risks and are costly to remove from drinking water. Their #CleanUpPFAS campaign advocates for significantly lower PFAS limits.[xxviii] These long-lasting chemicals, which are present in numerous products, require complex and costly removal technologies from water sources, as described in the literature. Chemical pollution causes significant damage to ecosystems and contributes to the decline of biodiversity. For example, a Studie shows that pesticides have severe negative effects on the biodiversity of stream invertebrates in both Europe and Australia. Research indicates that pesticides can lead to declines in up to 42% of the species pools of stream invertebrates. This level of decline is comparable to other major drivers of biodiversity loss.[xxix]Given these enormous environmental and economic costs, it is crucial to take decisive action to reduce chemical pollution. A comprehensive global approach is needed to protect the environment and health and secure a sustainable future for generations to come.
2.2 Measuring Firms’ Sustainability Performance Through KPI’s
In today’s era, where the public is increasingly focused on chemical pollution, companies face the challenge of accurately measuring and reducing their environmental impact. Key Performance Indicators (KPI’s) are essential tools for assessing and monitoring environmental performance, particularly with regard to chemical pollution. They allow companies to quantify their progress in reducing harmful emissions and waste and to implement targeted actions to improve their environmental record. In the long term, addressing chemical pollution is worthwhile for companies, as responsible corporate governance not only enhances their environmental performance but also brings economic benefits.
The following table presents a selection of KPIs used to measure chemical pollution:
Direct Toxicity Assessment (DTA) = used to assess the impact of chemical pollution on the environment, specifically evaluating the negative effects of unknown contaminants, often present as chemical mixtures, on aquatic and terrestrial ecosystems.[xxx] |
Hazardous Waste = measures the quantity and concentration of hazardous chemicals released during waste management and ensures compliance with EU regulations to protect the environment and human health.[xxxi] |
Compliance with Chemical Regulations = Tracks how well a company adheres to legal requirements and environmental standards concerning chemical substances, such as the REACH regulation in the EU or the Toxic Substances Control Act (TSCA) in the US.[xxxii] [xxxiii] |
Chemical Safety Incidents = measure the occurrence and severity of accidents involving hazardous substances to assess and improve safety, environmental protection, and response effectiveness.[xxxiv] |
Water Quality Index for Chemical Pollutants (WQI) = simplifies complex water quality data into a single, easy-to-understand value, reflecting the chemical pollution level across various water sources and uses.[xxxv] |
These KPIs are designed to measure and manage the environmental impact of chemical pollution. By applying these KPIs, companies can take targeted actions and achieve their sustainability goals.
2.3 Pro & Cons Key performance indicators
2.3.1 Direct Toxicity Assessment
Direct Toxicity Assessment (DTA) is a method for evaluating the direct toxic effects of chemical contaminants on environmental samples. Unlike traditional methods that focus on individual chemicals, DTA captures the cumulative toxic effects of complex chemical mixtures.30 DTA offers several advantages in evaluating environmental contamination. It captures the overall toxicity of chemical mixtures, which is crucial given that environmental pollutants often consist of complex mixtures. DTA supports environmental management by aiding in the risk assessment and management of contaminated water, soil, and waste samples.30 Additionally, it provides valuable insights into toxic effects that might not be detected through chemical analysis alone. However, DTA also has its drawbacks. The method can be expensive and complex, which may limit its application on a large scale. Furthermore, obtaining reliable toxicological data for all possible contaminants can be challenging, potentially leading to inaccuracies in the assessment.
2.3.2 Hazardous Waste
Hazardous Waste KPIs are vital for assessing the quantity and concentration of hazardous chemicals released during waste management, and for ensuring adherence to EU regulations aimed at protecting the environment and human health.31 For instance, data from the Federal Statistical Office (Destatis) indicate that in 2019, Germany generated 23.9 million tons of hazardous waste, marking a slight increase from the previous year. This total includes significant contributions from construction and demolition wastes (40%), waste from waste management facilities and public wastewater treatment (28%), and oil and liquid fuel wastes (6%).[xxxvi] Monitoring these KPIs is crucial for evaluating and enhancing waste management practices, mitigating risks, and ensuring compliance with environmental regulations.
KPIs for hazardous waste are crucial for assessing the quantity and concentration of hazardous chemicals released during waste management and ensuring compliance with EU regulations that protect the environment and human health. One advantage of these KPIs is that they help ensure adherence to legal requirements and contribute to minimizing risks to both the environment and public health. Additionally, they enable the optimization of waste management practices by identifying and addressing weaknesses. However, collecting the necessary data can be complex and resource-intensive, and there is a risk of inaccuracies in data analysis, which could affect the effectiveness of risk reduction measures.
2.3.3 Compliance with Chemical Regulations
Tracking compliance with chemical regulations is essential for ensuring that companies adhere to legal requirements and environmental standards regarding chemical substances. Key regulations include the EU Registration, Evaluation, Authorization, and Restriction of Chemicals (REACH) and the US Toxic Substances Control Act (TSCA). These regulations mandate comprehensive documentation, reporting, and safe handling of chemicals to protect human health and the environment.32 33
Monitoring compliance provides several benefits. It ensures that companies meet legal obligations, which helps avoid regulatory penalties and fosters public trust. Additionally, it supports effective environmental management by preventing chemical releases that could harm ecosystems and human health. However, the process can be complex and resource-intensive, requiring detailed tracking and reporting. Challenges may include managing large volumes of data and ensuring accuracy in compliance assessments, which can impact the overall effectiveness of regulatory adherence.
2.3.4 Chemical Safety Incidents
Measuring hazardous substance incidents tracks the frequency and severity of accidents involving dangerous materials to assess and enhance safety, environmental protection, and response effectiveness.34 These KPIs are essential for identifying vulnerabilities in handling hazardous substances, improving safety protocols, and refining emergency response plans.
One advantage of these KPIs is their role in optimizing safety measures and emergency strategies, which helps reduce environmental damage and health risks. Analyzing incident data also supports continuous improvement in response capabilities and safety practices. However, collecting and analyzing incident data can be complex and resource-intensive. Inaccurate or incomplete data may lead to inadequate safety measures and reduced response effectiveness, impacting the overall effectiveness of safety strategies.
2.3.5 Water Quality Index for Chemical Pollutants
The Water Quality Index (WQI) simplifies complex water quality data into a single, easily understandable value that reflects the level of chemical pollution across various water sources and uses.35 This KPI is crucial for providing a clear and unified overview of water quality, making it easier for stakeholders to identify and address contamination issues.
The main advantage of the WQI is its ability to present intricate water quality data in an accessible format, which facilitates communication and decision-making regarding water safety. It supports the monitoring and comparison of water quality across different sources and time periods. However, this simplification can result in the loss of detailed information and potential oversight of specific pollution issues. Additionally, the accuracy of the WQI depends on the quality and completeness of the underlying data, which can be challenging to collect and maintain.
2.4 Best Practice Examples
Dow Chemical has been a pioneer in the field of real-time air monitoring, setting up best practices for measuring chemical pollution. One of the primary methods employed involves the use of automatic analyzers, which provide high-resolution data on pollutant concentrations at specific points.[xxxvii] These analyzers can produce continuous measurements, which are essential for regulatory compliance and effective environmental management.[xxxviii] A significant advantage of Dow Chemical’s approach is the utilization of low-cost air quality analyzers. These devices are lightweight, portable, and easy to operate, enabling widespread deployment even in areas with limited resources. Additionally, passive sampling methods enhance spatial resolution and simplify the monitoring process, allowing for more comprehensive data collection in various environments.[xxxix] Dow Chemical also incorporates cutting-edge physical and physicochemical measuring techniques in their monitoring systems. These methods provide better stability, sensitivity, and reliability, which are crucial for detecting pollutants accurately. the practical application of these techniques requires minimal maintenance, making them ideal for continuous monitoring scenarios. By combining physical property measurements with chemical reactions, Dow Chemical achieves a comprehensive understanding of air quality.38
BASF, a global leader in chemical production, has implemented integrated water management and pollution tracking practices as a best practice model for addressing chemical pollution. This approach hinges on the substitution of harmful chemical products with less toxic, environmentally friendly alternatives. By training employees in the proper use of these products, BASF ensures that retailers are incentivized to promote them, thereby aiding in the reduction of urban non-point source pollution.[xl] One of the key components of BASF’s strategy is the use of best management practices (BMPs) to control non-point source pollution. BMPs such as buffer strips, fertilizer reduction, and increasing forest and grassland areas have been shown to effectively reduce the total nitrogen (TN) and total phosphorus (TP) loads in watersheds. Additionally, integrated assessment frameworks and decision support systems (DSS) are employed to evaluate the ecological and economic impacts of these BMPs, ensuring that the measures taken are both effective and cost-efficient.
3 Sustainability Implementation
Improving the sustainability performance of firms in the context of chemical pollution through multifaceted approaches that incorporate specific processes, tools and measures is essential. One significant approach is pollution prevention (P2). P2 is a proactive approach aimed at reducing or eliminating waste and pollutants at their source rather than managing them post-production. This method is crucial in the fight against chemical pollution as it tackles the problem at its origin. By redesigning processes, substituting materials, and improving operational practices, industries can significantly cut down on the generation of harmful chemicals and pollutants.[xli]
A key element of P2 is the Pollution Prevention Opportunity Assessment (P2OA), which systematically evaluates waste-generating activities to identify opportunities for waste reduction and material substitution.41 These assessments often involve modifying existing processes or adopting new technologies that are less polluting. For example, many industries have switched to using less hazardous materials or have implemented recycling systems to manage waste more efficiently. Such strategies not only reduce the environmental footprint but can also lead to cost savings and improved regulatory compliance.[xlii]
One notable example of a company successfully implementing P2 practices is the Idaho National Laboratory (INL). INL has integrated P2 into its core operations, focusing on both cultural and technical objectives to foster an environmentally conscious workplace. The lab promotes resource conservation, waste reduction, and the use of environmentally friendlymaterials. By conducting regular P2OAs, INL identifies and prioritizes opportunities for reducing toxic chemical use and enhancing recycling efforts. This comprehensive approach has helped INL not only minimize its environmental impact but also improve operational efficiency and mission sustainability. [xliii]
The adoption of pollution prevention activities (PPAs) is an important part of the boarder goal of P2. These activities emphasize on minimizing waste generation at its source, rather than relying on end-of-pipe treatments. Activities such as increasing the frequency of planned maintenance and adjusting batch schedules to reduce waste, have been shown to enhance resource-use efficiency and reduce production costs.[xliv] The implementation of pollution prevention strategies requires a detailed understanding of both the technical and financial aspects of proposed changes. Companies must evaluate the potential environmental benefits alongside the financial viability of these innovations. For example, the use of granular activated carbon biofilms in industrial wastewater treatment has been shown to be effective in removing harmful substances, demonstrating the potential for significant environmental improvements.[xlv] However, the decision to adopt such technologies often hinges on a thorough financial analysis to ensure that the benefits outweigh the costs.[xlvi] Worker involvement is another crucial element in the success of pollution prevention initiatives. Employees who manage and operate production processes daily possess valuable insights into how changes will impact efficiency and safety. Engaging workers in decision-making can lead to more effective and practical solutions. Their hands-on experience is particularly beneficial in complex systems where both the problems and solutions are not easily defined. A collaborative approach ensures that pollution prevention measures are not only technically sound but also feasible and sustainable in the long term.[xlvii] Companies such as DuPont have demonstrated that investing in pollution prevention can yield both environmental benefits and cost savings. DuPont’s modification of its production processes resulted in a 50% reduction in pollutant discharges and substantial cost savings, proving that environmental and financial objectives can be aligned.44
Another vital process is the implementation of Life Cycle Assessment (LCA) methodologies. LCA allows firms to evaluate the environmental impacts of chemicals throughout their entire life cycle, from raw material extraction to disposal. This comprehensive approach identifies stages where significant environmental harm occurs and helps in prioritizing areas for improvement. [xlviii] Understanding and mitigating the environmental footprint at each stage of the product life cycle, helps firms make more informed decisions and implement practices that reduce their overall impact on the environment, which can eventually lead to long-term improvements in both environmental and financial performance.49 Also integrating LCA with Environmental Risk Assessment (ERA) helps firms in better understanding the long-term impacts of their chemical use, ensuring that their practices do not exceed the carrying capacity of ecosystems.[xlix] This combined methodology offers a robust framework for assessing and mitigating the ecological footprint of chemical activities.
The integration of Green Chemistry is another effective tool which aims to create products and processes that are inherently non-polluting, thereby reducing the risk associated with chemical exposures.[l] The field of Green Chemistry focuses on designing chemical processes and products that minimize or eliminate the use and generation of hazardous substances. The introduction of the E Factor in 1992 marked a significant shift in how efficiency in chemical processes is measured, moving from a focus solely on chemical yield to the importance of waste elimination.[li] One of the fundamental principles of Green Chemistry is the use of renewable feedstocks instead of depleting natural resources. This principle aims to create a sustainable supply chain that reduces the dependency on non-renewable resources and minimizes environmental impact. [lii] Green Chemistry also promotes the use of safer solvents and reaction conditions, which helps to prevent the release of toxic chemicals into the environment. For example, replacing traditional organic solvents with water or solvent-free processes significantly reduces the risk associated with hazardous chemical exposures.[liii] Another aspect of Green Chemistry is the emphasis on catalysis over stoichiometric reagents. Catalysts can accelerate chemical reactions without being consumed in the process, leading to fewer by-products and less waste. This approach not only improves the efficiency of chemical processes but also aligns with the principle of atom economy, where all materials used in the process are maximized in the final product. [liv] Furthermore, real-time analysis and monitoring techniques are encouraged to ensure that processes remain within safe and sustainable parameters, thereby minimizing potential accidents and further reducing pollution.52
The principles of Green Chemistry have been widely adopted in various industries, particularly in pharmaceuticals and fine chemicals, where waste generation is a big concern. One exemplary company that has made significant strides in Green Chemistry is Pfizer. Recognizing the environmental impact of traditional synthetic routes, Pfizer redesigned its manufacturing processes for drugs such as sertraline hydrochloride and pregabalin. The green synthesis of these drugs has led to substantial reduction in waste and the use of hazardous solvents. Pfizer reported that the company eliminated nearly 38 million liters of alcoholic organic solvents annually by employing biocatalysts like lipase in the synthesis of pregabalin. [lv]
Integrated Pollution Prevention and Control (IPPC) is another comprehensive approach aimed at minimizing pollution from various industrial sources. One of the core principles of IPPC is to treat effluents and emissions at the source to prevent harmful substances from entering the environment. This involves the use of primary, secondary, and tertiary treatment methods for wastewater, as well as advanced technologies like membrane filtration and incineration to recover water and other materials from industrial effluents.[lvi] Additionally, the integration of chemical pollution research with ecological studies is essential for a holistic understanding of pollution’s impact on biodiversity. This integration is currently lacking, as chemical pollution research often remains siled within its own discipline, disconnected from broader ecological studies. Bridging this gap requires collaborative efforts among researchers from various fields, including ecotoxicology, environmental chemistry, and ecology. Such interdisciplinary collaboration can lead to more effective mitigation strategies that are grounded in a comprehensive understanding of ecosystem processes and chemical exposure effects.[lvii]
Political and regulatory frameworks also play a crucial role in the successful implementation of IPPC. The European Union’s Zero Pollution Ambition and the United Nations’ initiatives to manage chemicals and waste reflect a growing political awareness of the need to address chemical pollution on a global scale. These frameworks emphasize the importance of sound science-policy interfaces, similar to those established for climate change and biodiversity. By setting clear regulatory targets and fostering international cooperation, these initiatives aim to create an environment where scientific research can effectively inform policy decisions and lead to tangible environmental improvements. Despite these advancements, challenges remain in achieving effective IPPC. One significant hurdle is the lack of standardized and reliable analysis methods for detecting hazardous chemicals in the environment. Accurate measurements and monitoring are critical for risk assessment and regulatory compliance. Therefore, ongoing efforts to develop and standardize high-sensitivity analytical techniques are vital for the long-term success of IPPC initiatives.[lviii]57
Another tool in the context of implementing chemical pollution sustainability is the Best Available Techniques Framework (BAT). This framework aims to minimize environmental impacts and reduce emissions and waste by utilizing the most effective methods and practices available, often tailored to specific sectors and processes. The implementation of BAT involves a thorough assessment of available technologies, focusing on their technical and economic viability. In the textile industry, studies have shown that membrane filtration processes, which include microfiltration (MF) and nanofiltration (NF), are the preferred BAT for water recovery from dyeing wastewater due to their lower environmental impact and cost-effectiveness compared to alternatives like ozonation.62
In the Nordic foundry sector, BAT includes techniques such as the use of bag-house filters and pressure drop gauges to control air emissions, as well as optimized processes for chemical handling and substitution to minimize environmental impacts.[lix] One critical aspect of BAT is its focus on the entire lifecycle of industrial processes, from raw material selection to waste management. For instance, in wood preservation with chemicals, BAT methodologies emphasize the control and optimized consumption of chemicals to avoid accidental spills and releases into the environment. Regular maintenance of equipment and the use of less harmful chemicals when possible are also recommended. These measures not only protect the environment but also improve operational efficiency and reduce costs associated with waste treatment and disposal.[lx]
To ensure the effective application of BAT, it is crucial to have a clear and user-friendly assessment methodology. The L-BAT (Local-BAT) approach, for instance, provides a structured framework for industries and authorities to evaluate and justify the use of BAT at the local level. This method incorporates multiple criteria, including technical operations, management policies, and local environmental conditions, to create a comprehensive decision-support tool.[lxi] BAT is endorsed by the IPPC directive, which sets out common rules for industrial activities with significant pollution potential. This directive emphasizes the importance of using low-waste technology, energy-efficient processes, and measures to minimize the risk of environmental hazards.[lxii]
ISO 14001 certification is another critical tool for promoting chemical pollution sustainability. This international standard provides a framework for organizations to develop and implement effective Environmental Management Systems (EMS). The adoption of ISO 14001 involves a Plan-Do-Check-Act methodology, which helps companies systematically manage their environmental responsibilities, ensuring compliance with regulations and promoting continuous improvement.[lxiii]By adhering to this standard, firms can not only enhance their environmental performance but also build a positive image among stakeholders and regulators.[lxiv] The certification process encourages organizations to identify and control their environmental impacts, set performance objectives, and monitor progress. Companies that achieve ISO 14001 certification often experience benefits such as reduced waste, lower energy consumption, and improved corporate reputation. The primary motivations for companies to seek ISO 14001 certification include a sense of environmental responsibility, the desire to be seen as socially responsible, and the aim to improve overall performance. Research indicates that these motives often translate into tangible benefits, such as reduced waste, improved efficiency, and better profitability.[lxv] Furthermore, companies that are ISO 14001 certified tend to have better compliance records with environmental regulations compared to non-certified firms, which highlights the certification’s role in fostering regulatory adherence.[lxvi] However, the effectiveness of ISO 14001 in significantly reducing pollution and enhancing environmental performance is a subject of debate. While some studies have found that ISO 14001 certification leads to improved environmental outcomes, such as reduced waste and emissions, others argue that the certification alone does not guarantee superior environmental performance. The variation in the quality of implementation among certified firms suggests that without rigorous adherence to the standard’s requirements, the certification may serve more as a symbolic gesture rather than an effective tool for environmental improvement.63
The following Figure 1: “Overview of Sustainability Processes and Tools regarding Chemical Pollution” visualizes the previously mentioned processes and tools.
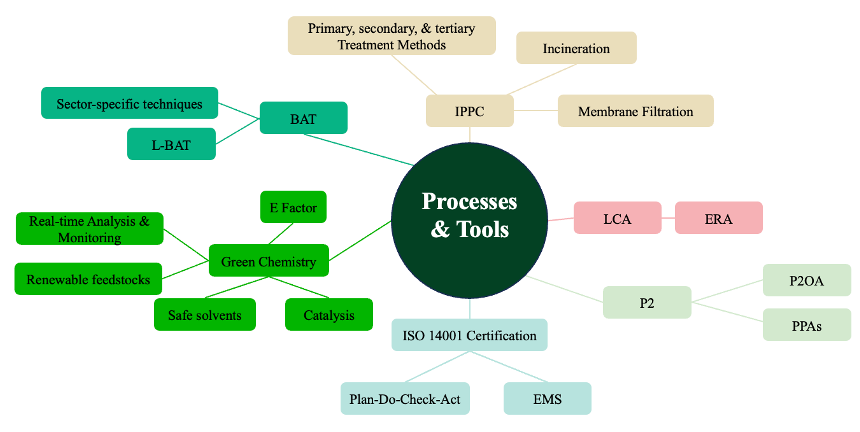
The following table 3 shows the main advantages and disadvantages of each of the tools and processes mentioned above.
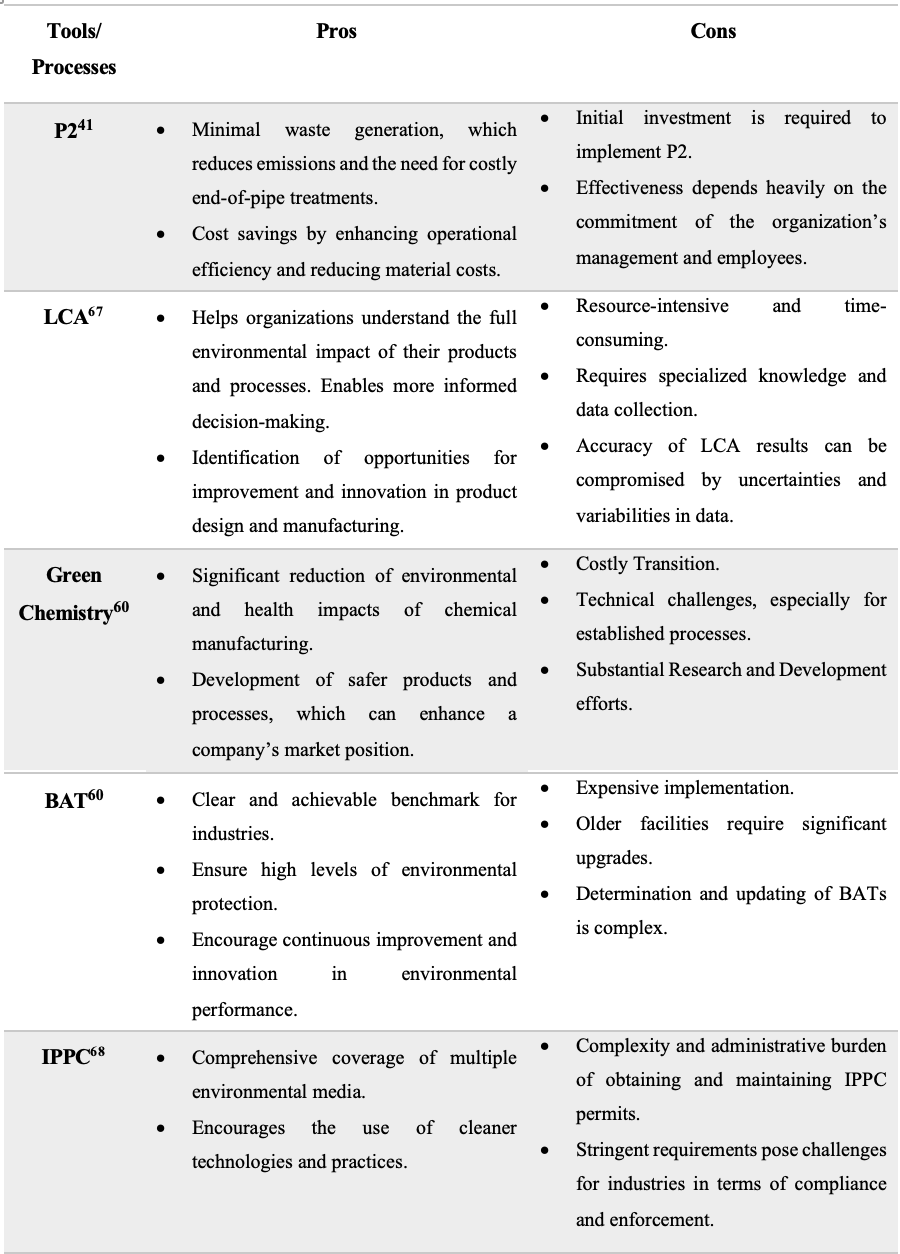
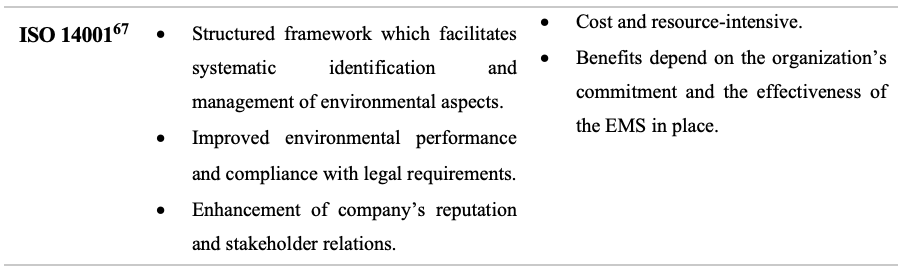
4 Drivers and Barriers
4.1 Drivers
When it comes to chemical pollution, sustainability is driven by various factors that collectively aim to reduce environmental harm and promote ecological balance. Each of these factors plays a crucial role in mitigating the adverse effects on both the environment and human health. One significant driver is the development and implementation of stringent environmental regulations. For instance, the Control of Major Accident Hazard (COMAH) Regulations in the UK emphasize the importance of proactive measures in managing chemical hazards, underscoring the need for collaboration between health and safety advisers, occupational physicians, and local health authorities.[lxix] Also regulations like REACH in the European Union require the registration, evaluation, authorization, and restriction of chemicals, ensuring that harmful substances are identified and managed properly.[lxx] Regulations such as the EU-Water Framework Directive (WFD) and the U.S. Clean Water Rule play crucial roles in maintaining water quality by limiting the levels of harmful chemicals in surface waters. These regulations not only enforce compliance but also encourage industries to adopt safer practices and technologies to reduce chemical emissions and promote the development of safer alternatives.
Economically, the costs associated with chemical pollution are substantial and multifarious. Firms may incur direct costs from fines and remediation efforts, as well as indirect costs like reputational damage and reduced consumer trust. Moreover, pollution can affect a firm’s bottom line by triggering health issues among workers and local communities, leading to decreased productivity and increased healthcare expenses. The economic burden extends to the broader economy, as contaminated natural resources can hinder sectors like agriculture and tourism, ultimately affecting national economic health. Due to this, firms can be driven from an economical perspective to invest in more sustainable solutions.[lxxi]
Socially, chemical pollution has far-reaching implications for communities, particularly in middle- and low-income countries. In regions like Eastern Africa, the release of chemical pollutants into the environment is linked to significant public health crises, causing diseases and fatalities, especially among vulnerable populations such as children.[lxxii] The social fabric of these communities is strained as public health deteriorates, leading to increased grievances and subsequent activism. This activism, in turn, pressures firms and governments to take more robust actions against pollution.71
Technological advancements, particularly in catalysis and carbon capture and utilization (CCU), also play a crucial role. Catalysis helps in increasing the efficiency of chemical reactions, reducing waste and energy consumption. Moreover, CCU technologies have shown potential in reducing the global warming impact by converting CO2 into useful products, such as formic acid and polyols, which are less harmful to the environment.[lxxiii] These advancements are essential for creating a sustainable industrial ecosystem that emulates natural systems where waste is minimized, and resources are efficiently utilized. Also, innovations in bioremediation, which utilize microorganisms and plants to neutralize pollutants, offer eco-friendly and cost-effective solutions.[lxxiv] In addition, the development of predictive and retrospective tools for assessing chemical impacts can help firms better manage their environmental footprint. Technologies such as micro-reactions and solvent-free synthesis further enhance resource efficiency and reduce emissions.76 These methods significantly reduce the use of hazardous solvents and minimize waste, contributing to cleaner production practices. The push towards such green chemistry techniques is supported by the need to comply with stringent water quality regulations like the WFD and the Clean Water Rule. For example, the WFD requires member states to monitor and assess the chemical status of water bodies, encouraging industries to adopt cleaner technologies to meet these standards.[lxxv]Another driver is sustainable chemistry, which involves designing chemicals and processes that are inherently safe and environmentally friendly. This includes the development of catalysts that enhance reaction efficiency and the use of biobased raw materials to replace fossil resources.[lxxvi]
On a firm-internal level, it is essential to consider the role of different departments. The research and development (R&D) department within firms represents another driver. R&D teams are pivotal in developing safer chemicals and more efficient production processes. Firms that prioritize R&D in green chemistry can achieve significant reductions in pollution.[lxxvii] The production department is often motivated to maximize output and minimize costs, which can sometimes lead to the adoption of less environmentally friendly practices. However, when firms invest in pollution-reduction technologies, the production department may benefit from streamlined processes and reduced waste, potentially leading to cost savings in the long run.78
Additionally, Human resources (HR) departments also play a key role in influencing a firm’s approach to chemical pollution. Companies with a more educated workforce tend to have lower pollution levels, as educated employees are more likely to be aware of and adhere to environmental regulations. HR departments can drive positive change by prioritizing the hiring of educated personnel and providing ongoing training on environmental best practices.[lxxviii]
The corporate governance and compliance department within firms plays a substantial role in ensuring that firms adhere to environmental regulations. Publicly listed firms, which are subject to greater scrutiny from investors and regulators, often demonstrate better compliance with pollution control measures compared to state-owned enterprises. Compliance departments must navigate the complexities of environmental regulations, balancing the need to meet legal requirements with the operational demands of the business. However, inconsistent enforcement of regulations and local protectionism can undermine these efforts, particularly in regions where economic growth is prioritized over environmental protection.78
4.2 Barriers
A key external barrier faced by firms is the complexity and uncertainty of regulatory requirements. For instance, some companies find it difficult to navigate the myriad of environmental regulations, which can vary significantly across regions and sectors.[lxxix] To overcome these barriers, firms have successfully adopted strategies such as engaging in proactive communication with regulatory bodies and participating in industry consortia. These efforts not only help firms stay informed about regulatory changes but also enable them to influence policy development in a way that aligns with their operational capabilities.
Another external barrier is the perception of financial markets towards firms’ environmental investments. An important barrier for firms is the initial cost of implementing PPAs. Despite the positive impact of PPAs on operational costs, these activities do not always translate into immediate market valuation gains. Studies have shown that while intensive PPAs improve cost competitiveness, they do not necessarily lead to higher market valuations. This indicates a disconnect between environmental performance and market perception. To bridge this gap, firms need to communicate the long-term financial benefits of their environmental practices effectively to investors and stakeholders. By doing so, they can align market expectations with their sustainable development goals, ultimately fostering a more supportive external environment for their pollution prevention efforts.44
On a firm-internal level, the previously mentioned drivers can also be presented as barriers and bring challenges. R&D efforts, for instance, require substantial investment and a long-term perspective, which can be a barrier for firms focused on short-term financial performance. Additionally, the lack of immediate tangible benefits from R&D in green chemistry can deter firms from investing in these essential activities.77 When it comes to the role of HR departments attracting and retaining highly educated employees can be a challenge, particularly for smaller firms or those in highly competitive markets where salary and benefits packages may be less attractive.78 Investing in pollution-reduction technologies in the production department carries the barrier of the initial high costs of installing these technologies, which can be a significant barrier, particularly for smaller firms with limited financial resources.[lxxx] Furthermore, although corporate governance and compliance efforts can ensure that firms adhere to environmental regulations, an inconsistent enforcement of regulations and local protectionism can undermine these efforts, particularly in regions where economic growth is prioritized over environmental protection.78 Additionally, many firms focus primarily on environmental reporting rather than actual corporate practices, which can lead to a superficial engagement with environmental issues and hinder meaningful progress. This lack of operationalization can result in missed opportunities for genuine environmental improvements.[lxxxi]
Firms must address both firm-external and firm-internal barriers in order to effectively prevent pollution. An essential internal strategy for overcoming barriers is fostering a culture of environmental responsibility within the organization. Many firms have achieved this by engaging employees in environmental initiatives. For instance, internal team management, employee engagement, and internal audits have been reported as common sources of PPAs within the chemical industry. When employees are actively involved in environmental practices, it leads to more innovative solutions and a collective effort towards reducing pollution. This approach not only enhances environmental performance but also improves cost efficiency by optimizing resource use and minimizing waste.44 Externally, firms often face regulatory pressures and market expectations. California’s Green Chemistry laws provide an excellent example of how regulatory frameworks can drive firms towards better practices. Ten years after these laws were enacted, an evaluation showed that they significantly improved transparency, protected vulnerable populations, and placed the burden of compliance on manufacturers, thus promoting safer chemical use. This regulatory push not only helped in reducing pollution levels but also encouraged firms to adopt greener and more sustainable practices, demonstrating the power of well-structured policies in overcoming external barriers.[lxxxii]
References
1Naidu, R., et al. Chemical pollution: A growing peril and potential catastrophic risk to humanity. Environment International 156, 1-3 (2021).
[ii]Diamond, M. L., et al. Exploring the planetary boundary for chemical pollution. Environment International 78, 9 (2015).
[iii] Sial, J. K. et al. (2022). Water Pollution from Agriculture and Industry. International Journal Of Current Engineering And Technology 12(03), 244-247 (2022).
[iv] Sigmund, G., et al. Addressing chemical pollution in biodiversity research. Global Change Biology 29(12), 3242 (2023).
[v] Dreyer, A. & Minkos, A. Polychlorinated biphenyls (PCB) and polychlorinated dibenzo-para-dioxins and dibenzofurans (PCDD/F) in ambient air and deposition in the German background. Environmental Pollution 316, 120511, 1-3 (2023).
[vi]BVL – Stockholmer Übereinkommen (POP-Konvention). https://www.bvl.bund.de/DE/Arbeitsbereiche/04_Pflanzenschutzmittel/03_Antragsteller/13_Rechtsvorschriften/03_intern_abk/03_pop/psm_intern_abk_pop_node.html(2023).
[vii] Kumar, V. et al. Removal of Inorganic Pollutants from Wastewater: Innovative Technologies and Toxicity Assessment. Sustainability 15(16376), 3 (2023).
[viii] Kaur, R., et al. Pesticides: An alarming detrimental to health and environment. Science of the Total Environment 915, 170113, 2-23 (2024).
[ix] Kiran, R., Bharti, R., & Sharma, R.. Effect of heavy metals: An overview. Materials Today: Proceedings 51, 880–885 (2021).
[x] Chen, D., et al. The Vertical Distribution of VOCs and Their Impact on the Environment: A Review. Atmosphere 13, 1940, 1-2 (2022).
[xi] Zhou, X., Zhou, X., Wang, C. & Zhou, H. Environmental and human health impacts of volatile organic compounds: A perspective review. Chemosphere 313(137489), 2-7 (2023).
[xii] Habib, Z., Song, M., Ikram, S. & Zahra, Z. Overview of Per- and Polyfluoroalkyl Substances (PFAS), Their Applications, Sources, and Potential Impacts on Human Health. Pollutants 4, 137–146 (2024).
[xiii] EPA. Nutrient Pollution. Sources and Solutions: Agriculture. https://www.epa.gov/nutrientpollution/problem (2024).
[xiv] Isiuku, B. O. & Enyoh, C. E. Pollution and health risks assessment of nitrate and phosphate concentrations in water bodies in South Eastern, Nigeria. Environmental Advances 2(100018) (2020).
[xv] Persson, L. et al. Outside the Safe Operating Space of the Planetary Boundary for Novel Entities. Environmental Science & Technology 56, 1510–1511. (2022).
[xvi] Rockström, J., et al. A safe operating space for humanity. Nature 461, 472–475 (2009).
[xvii] Brooks, B. W., et al. Toxicology Advances for 21st Century Chemical Pollution. One Earth 2(4), 312 (2020).
[xviii] Gabrielli, P., et al. Net-zero emissions chemical industry in a world of limited resources. One Earth 6, 682 (2023).
[xix] IEA. Technology Roadmap: Energy and GHG Reductions in the Chemical Industry via Catalytic Processes.https://www.iea.org/reports/technology-roadmap-energy-and-ghg-reductions-in-the-chemical-industry-via-catalytic-processes (2013).
[xx] Filho, W. L. et al. An overview of the contribution of the textiles sector to climate change. Frontiers in Environmental Science 10, 1-2 (2022).
[xxi]Zhou, B. & Li, X. The monitoring of chemical pesticides pollution on ecological environment by GIS. Environmental Technology & Innovation 23, 2–4 (2021).
[xxii]Ganesh, K. N., et al. Green Chemistry: A Framework for a Sustainable Future. The Journal of Organic Chemistry 86, 8552 (2021).
[xxiii] Diaz, R. J. & Rosenberg, R. Spreading dead zones and consequences for marine ecosystems. Science 321, 926 (2008).
[xxiv] Oaks, J. L. et al. Diclofenac residues as the cause of vulture population decline in Pakistan. Nature 427, 1-4 (2004).
[xxv] World Health Organization. Lead poisoning and health. https://www.who.int/news-room/fact-sheets/detail/lead-poisoning-and-health (2023).
[xxvi] Lanphear, B. P., et al. Low-level lead exposure and mortality in US adults: a population-based cohort study. The Lancet Public Health 3(4), e180–e182 (2018).
[xxvii] Emerging chemical risks in Europe — ‘PFAS’.European Environment Agency https://www.eea.europa.eu/ publications/emerging-chemical-risks-in-europe (2023).
[xxviii] Royal Society of Chemistry. (o. D.). RSC challenges UK Government to reduce PFAS levels in British water as research highlights serious health risks posed by ‘forever chemicals’ https://www.rsc.org/news-events/ articles/2023/oct/pfas-cleaning-up-uk-drinking-water/ (2023).
[xxix] Beketov, M. A., Kefford, B. J., Schäfer, R. B. & Liess, M. Pesticides reduce regional biodiversity of stream invertebrates. Proceedings of the National Academy of Sciences 110, 11039–11043 (2013).
[xxx] Gruiz, K. et al. Direct toxicity assessment — Methods, evaluation, interpretation. Science of the Total Environment 563–564, 803–812 (2016).
[xxxi] Hazardous waste. Umweltbundesamt. https://www.umweltbundesamt.de/en/topics/waste-resources/waste- management/waste-types/hazardous-waste (2014).
[xxxii] Herrmann, A. & Maeß, C. Die Chemikalien‐Verordnung REACH. Chemie In Unserer Zeit 54, 30–43 (2019).
[xxxiii] Lynch, H. N., Allen, L. H., Hamaji, C. M. & Maier, A. Strategies for refinement of occupational inhalation exposure evaluation in the EPA TSCA risk evaluation process. Toxicology and Industrial Health 39, 169–182 (2023).
[xxxiv] Industrial accidents. Environment. https://environment.ec.europa.eu/topics/industrial-emissions-and-safety/industrial-accidents_en#implementation (2024).
[xxxv] Syeed, M. M. M. et al. Surface water quality profiling using the water quality index, pollution index and statistical methods: A critical review. Environmental And Sustainability Indicators 18, 100247, 1-23 (2023).
[xxxvi] 23.9 million tonnes of hazardous waste in 2019. Federal Statistical Office. https://www. destatis.de/EN/Press/2021/07/PE21_348_321.html (2024).
[xxxvii] Ionel, I., & Popescu, F. Methods for Online Monitoring of Air Pollution Concentration. Sciyo, (2010).
[xxxviii] Namiesnik, J., Sadowski, J., Stefanski, J., & Wardencki, W. The Impact of Air Pollution on Health, Economy, Environment and Agricultural Sources Monitoring of Gaseous Air Pollution, InTech, (2011).
[xxxix] Cerrato-Alvarez, M., Samuel F., & Pinilla-Gil, E. Use of Uncertainty Calculation Software as a Didactic Tool to Improve the Knowledge of Chemistry Students in Analytical Method Validation. Journal of Chemical Education 101(1), 104–112 (2023).
[xl] Hayden,L., et al.. Residential Adoption of Best Landscape Management Practices: Effects of Outreach to Reduce Non-Point Source Pollution. Land 12 (2), 382 (2023).
[xli] Engel, J. A. Pollution prevention program implementation plan. United States, 1-20 (1996).
[xlii] Bi, X., Mullally, C., & Gaonkar, S. Does Peer Adoption Increase the Diffusion of Pollution Prevention Practices?. Land Economics 97(1), 224-245 (2021).
[xliii] Sellers, E. D. Idaho National Laboratory Site Pollution Prevention Plan. United States, 1-31 (2007).
[xliv] Yoo, S., Eom, J. & Han, I. Too Costly to Disregard: The Cost Competitiveness of Environmental Operating Practices. Sustainability 12 (15), 5971 (2020)
[xlv] Sil, A. et al. Strategies for mitigation of polychlorinated biphenyls (PCBs): A review. International Journal of Chemical Studies 8(4), 1700-1708 (2020).
[xlvi] Boyd, J. Searching for the Profit in Pollution Prevention: Case Studies in the Corporate Evaluation of Environmental Opportunities. Resources from the future, Discussion Paper 98-30 (1998).
[xlvii] Tickner, J. & Wright, S. Primary Prevention of Chemical Contamination. NEW SOLUTIONS: A Journal of Environmental and Occupational Health Policy 12(4), 425-433 (2003).
[xlviii] Van Aken, K., Strekowski, L., & Patiny, L. EcoScale, a semi-quantitative tool to select an organic preparation based on economical and ecological parameters. Beilstein journal of organic chemistry, 2(1), 3 (2006).
[xlix] Sala, S. & Saouter, E. Planetary Boundaries and Chemical Pollution: A Grail Quest?. Chemistry International, 36(6), 2-4 (2014).
[l] Cui, Z., Beach, E. & Anastas, P. Green chemistry in China. Pure and Applied Chemistry, 83(7), 1379-1390 (2011).
[li] Sheldon, R. A. The E factor at 30: a passion for pollution prevention. Green Chemistry 25(5), 1704-1728 (2023).
[lii] Mitralis, M., Azizah, U. & Yonata, B. The integration of green chemistry principles in basic chemistry learning to support achievement of Sustainable Development Goals (SDGs) through education. Journal of Technology and Science Education 13(1), 233-254 (2023).
[liii] Stubbs, S., Yousaf, S. & Khan, I. A review on the synthesis of bio-based surfactants using green chemistry principles. DARU Journal of Pharmaceutical Sciences 30, 407–426 (2022).
[liv] Ravichandran, V. Green Chemistry–An Environmentally Benign Chemistry. Journal of Young Pharmacists 10(2), 131 (2018).
[lv] Menges, N. The Role of Green Solvents and Catalysts at the Future of Drug Design and of Synthesis. Green Chemistry. (InTech, 2018)
[lvi] Kharat, D. S. Critical review of pollution control technologies. International Journal of Biosen Bioelectron 3(5), 338–340 (2017).
[lvii] Sylvester, F. et al. Better integration of chemical pollution research will further our understanding of biodiversity loss. Nature Ecology & Evolution 7, 1552–1555 (2023).
[lviii] Taniyasu, S., Hanari, N.,Horii, Y. & Yamashita, N. Standardization of environmental analysis methods of hazardous chemicals – contribution to international control of hazardous chemicals by using advanced technologies. Synthesiology 5(4), 261-276 (2012).
[lix] Wänerholm, M. Nordic Foundries : Best Available Techniques (BAT). (Copenhagen: Nordisk Ministerråd, 2017).
[lx] Salminen, E., Valo, R., Korhonen, M., & Jernlås, R. Wood preservation with chemicals: Best Available Techniques (BAT). (Copenhagen: Nordisk Ministerråd, 2014).
[lxi] Cikankowitz, A. & Laforest, V. How To Compare Industrial Techniques To Best Available Techniques? WIT Transactions on Ecology and the Environment, Vol 109, 707-716 (2008).
[lxii] Dogan, B., Kerestecioglu, m., Yetis, U. Assessment of the best available wastewater management techniques for a textile mill: cost and benefit analysis. Water Science & Technology 61(4), 963–970 (2010).
[lxiii] Aravind, D. & Christmann, P. Decoupling of Standard Implementation from Certification: Does Quality of ISO 14001 Implementation Affect Facilities’ Environmental Performance?. Business Ethics Quarterly 21(1), 73–102 (2011).
[lxiv] Riaz, H., Saeed, A., Baloch, M.S., Nasrullah & Khan, Z.A. Valuation of Environmental Management Standard ISO 14001: Evidence from an Emerging Market. Journal of Risk Financial Management 12(1), 1-14 (2019).
[lxv] Jovanovic, D. & Janjic, V. Motives For, Benefit From and Accounting Support to the ISO 14001 Standard Implementation. Economic Horizons 20(1), 25-41 (2018).
[lxvi] Potoski, M. and Prakash, A. Green Clubs and Voluntary Governance: ISO 14001 and Firms’ Regulatory Compliance. American Journal of Political Science 49(2), 235-248 (2005).
[lxvii] Ciravegna Martins da Fonseca, L. ISO 14001:2015: An improved tool for sustainability. Journal of Industrial Engineering and Management 8(1), 37-50 (2015).
[lxviii] Samarakoon, S. & Gudmestad, O.T. Air pollution control in a new oil and gas developments using best available techniques. WIT Transactions on Ecology and the Environment 136, 97-107 (2010).
[lxix] Palmer, S. R., Rees, H., & Coleman, G. Major chemical incidents: bridging the occupational–public health gap. Occupational medicine (Oxford, England) 50(4), 221–225 (2000).
[lxx] Fadel, C. & Tarabieh, K. Development of an Industrial Environmental Index to Assess the Sustainability of Industrial Solvent-Based Processes. Resources 8(2), 115 (2019).
[lxxi] van Rooij, B. The People vs. Pollution: understanding citizen action against pollution in China. Journal of Contemporary China 19(63), 55–77 (2010).
[lxxii] Cristiano, W., Giacoma, C., Carere, M., & Mancini, L. Chemical pollution as a driver of biodiversity loss and potential deterioration of ecosystem services in Eastern Africa: A critical review. South African Journal of Science 117(9/10), 30-36 (2021).
[lxxiii] Thonemann, N. & Pizzol, M. Consequential life cycle assessment of carbon capture and utilization technologies within the chemical industry. Energy & Environmental Science 12(7), 1-12 (2019).
[lxxiv] Egbo, T. E. et al. Strategies for Remediating Environmental Pollution, and Applicable Indicators for Identifying them: Mini Review. Biomedical Journal of Scientific & Technical Research 13(3), 9926-9935 (2019).
[lxxv] Undeman, E., Josefsson, H., Ågerstrand, M., Sobek, A. & Nilsson, A.. The potential of the EU Water Framework Directive for reducing emissions of pollutants is limited: a case study on river basin specific pollutants in Swedish environmental permitting processes. Environmental Sciences Europe 34 (1), 123 (2022).
[lxxvi] Hempel, M. Funding activities by the German Federal Environmental Foundation (Deutsche Bundesstiftung Umwelt) in the field of sustainable chemistry. Green Processing and Synthesis 1(3), 253-259 (2012).
[lxxvii] Schwarzman, M. R. & Wilson, M. P. New Science for Chemicals Policy. Science 326,1065-1066 (2009).
[lxxviii] Jiang, L., Lin, C. & Lin, P. “The determinants of pollution levels: Firm-level evidence from Chinese manufacturing,” Journal of Comparative Economics 42(1), 118-142 (2014).
[lxxix] Intergovernmental Panel on Climate Change (IPCC) (Ed.). National and Sub-national Policies and Institutions. In Climate Change 2022 – Mitigation of Climate Change: Working Group III Contribution to the Sixth Assessment Report of the Intergovernmental Panel on Climate Change. Cambridge: Cambridge University Press, 1355–1450 (2023).
[lxxx]Alberini, A. & Austin, D. Accidents Waiting to Happen: Liability Policy and Toxic Pollution Releases. The Review of Economics and Statistics 84 (4), 729–741 (2002).
[lxxxi] Jasni, N. S. & Yusoff, H. Key Factors of Successful Corporate Environmental Practice in Selected Malaysia Public Listed Companies. Environment-Behaviour Proceedings Journal 5(15), 19–25 (2020).
[lxxxii] Solomon, G. M., Hoang, A. & Reynolds P. The Califorfnia Safer Consumer Products Program: Evaluating a Novel Chemical Policy Strategy. NEW SOLUTIONS: A Journal of Environmental and Occupational Health Policy 29(2), 224-241 (2019).